Automated synthesis allows for discovery of charge transport in organic molecules
A cross-disciplinary UIUC team has demonstrated a major breakthrough in using automated synthesis to discover new molecules for organic electronics applications.
The technology that enabled the discovery relies on an automated platform for rapid molecular synthesis at scale—which is a game-changer in the field of organic electronics and beyond. Using automated synthesis, the team was able to rapidly scan through a library of molecules with precisely defined structures, thereby uncovering, via single-molecule characterization experiments, a new mechanism for high conductance. The work was just reported in Nature Communications and is the first major result to emerge from the Molecule Maker Lab, which is located in the Beckman Institute for Advanced Science and Technology at the University of Illinois Urbana-Champaign.
The unexpectedly high conductance was uncovered in experiments led by Charles M. Schroeder (BSD), who is the James Economy Professor in materials science & engineering and a professor in chemical & biomolecular engineering. The project’s goal was to seek out new molecules with strong conductivity that might be suitable for use in molecular electronics or organic electronics applications. The team’s approach was to systematically append many different side chains to molecular backbones to understand how the side chains affected conductance.
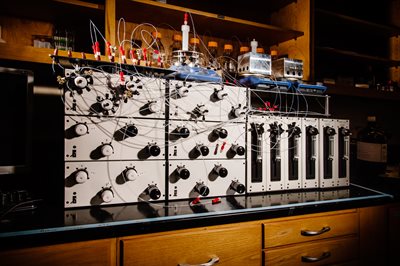
The first stage of the project consisted of synthesizing a large library of molecules to be characterized using single-molecule electronics experiments. If the synthesis had been done with conventional methods, it would have been a long, cumbersome process. That effort was avoided through use of the Molecule Maker Lab’s automated synthesis platform, which was designed to facilitate molecular discovery research that requires testing of large numbers of candidate molecules.
Edward R. Jira, a Ph.D. student in chemical & biomolecular engineering who had a leading role in the project, explained the synthesis platform’s concept. “What’s really powerful… is that it leverages a building-block-based strategy where all of the chemical functionality that we’re interested in is pre-encoded in building blocks that are bench-stable, and you can have a large library of them sitting on a shelf,” he said. A single type of reaction is used repeatedly to couple the building blocks together as needed, and “because we have this diverse building block library that encodes a lot of different functionality, we can access a huge array of different structures for different applications.”
As Schroeder put it, “Imagine snapping Legos together.”
Co-author Martin D. Burke (MMG) extended the Lego-brick analogy to explain why the synthesizer was so valuable to the experiments—and it wasn’t only because of the rapid production of the initial molecular library. “Because of the Lego-like approach for making these molecules, the team was able to understand why they are super-fast,” he explained. Once the surprisingly fast state was discovered, “using the ‘Legos,’ we could take the molecules apart piece by piece, and swap in different ‘Lego’ bricks—and thereby systematically understand the structure/function relationships that led to this ultrafast conductivity.”
Ph.D. student Jialing (Caroline) Li, an expert in single-molecule electronics characterization who studied the molecules generated by the synthesizer, explained the essence of the conductivity discovery. “We observed that the side chains have a huge impact on how the molecule behaves and how this affects charge transport efficiency across the entire molecule,” she said. Specifically, the team discovered that molecular junctions with long alkyl side chains have unexpectedly high conductance, which is dependent on concentration. They also figured out the reason for the high conductivity: the long alkyl side chains promote surface adsorption (the molecule’s ability to adhere to a surface), which results in planarization (in effect, flattening out) of the molecules such that electrons can flow through them more efficiently.
Burke, who is the May and Ving Lee Professor for Chemical Innovation and a professor of chemistry, called the building-block approach a “one-two punch”: it makes the platform “a powerful engine for both discovering function, and then understanding the function.”
The conductance discovery represents a significant advance for the field of organic electronics.
“Semiconductor-metal interfaces are ubiquitous in electronic devices. The surprising find of a high conductance state induced by metallic interfaces can pave the way to new molecular design for highly efficient charge injection and collection across a wide range of electronic applications,” said co-author Ying Diao, an I. C. Gunsalus Scholar, Dow Chemical Company Faculty Scholar, and associate professor of chemical & biomolecular engineering.
Schroeder explained that organic electronic materials have multiple benefits. To begin with, their use avoids the need for metals or other inorganic electronics. But organic electronics also offer much more: deformation and elastic properties that can be vital to some applications, such as implantable medical devices that could bend and flex along with, for example, a beating heart. Such organic devices could even be designed to degrade within the body, so that they break down and disappear after their job is done.
Some organic electronics are already available in commercial products. For example, organic light-emitting diodes (OLED) can be found in the screens of smart phones, smart watches, and OLED TVs. It’s anticipated that organic solar cells are on their way to becoming a commercial success as well. But the research community has only scratched the surface of organic electronics’ potential; progress has been slowed by the lack of key materials discoveries like the one just made by the UIUC team.
Schroeder said that it’s significant to have proven that “we can design and synthesize large libraries for various applications.” The paper “showcases the fact that we successfully did it for a class of molecules for molecular electronics.” He admitted, “I didn’t expect to see something as interesting on this first study!”
Co-author Jeffrey S. Moore (BSD), who is a Stanley O. Ikenberry Endowed Chair, professor of chemistry, and Howard Hughes Medical Institute Professor, reflected on the work: “Advancing basic science and technology by combining new facilities with a collaborative team is what makes the Beckman Institute so special. This discovery is the first of many that will come from the Molecule Maker Lab.”
Schroeder believes that the Molecule Maker Lab facilities—which also offer artificial intelligence capabilities for predicting what molecules are likely to be worth making—will open up a new approach to research in that “you can start thinking about designing based on a function instead of a structure.” Whereas researchers today might start by saying, “I need to make this particular structure because I think it’s going to do something,” it will be possible to tell the system, “I want to get this ultimate function,” and then let it help you figure out what structures you should make to get that function.
The intent is eventually to make the Molecule Maker Lab facilities available to researchers outside UIUC. Burke said he’d like to see the Lab “become a global epicenter of democratized molecular innovation,” empowering people who are not molecular synthesis specialists to solve important research problems.
“I think this is the beginning of something really special,” Burke said. “The journey has begun.”