By listening, scientists learn how a protein folds
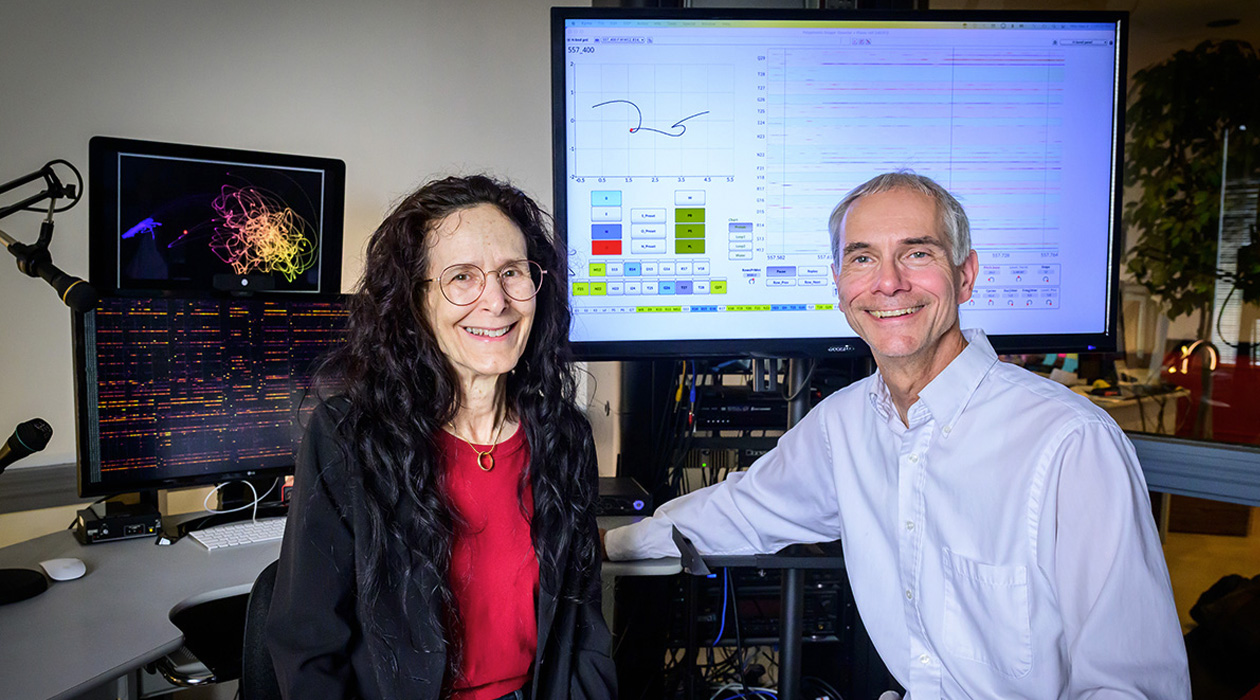
Composer and software developer Carla Scaletti and chemistry professor Martin Gruebele used sound to investigate hydrogen-bond dynamics during the protein-folding process. / Fred Zwicky
By converting their data into sounds, scientists discovered how hydrogen bonds contribute to the lightning-fast gyrations that transform a string of amino acids into a functional, folded protein. Their report, in the Proceedings of the National Academy of Sciences, offers an unprecedented view of the sequence of hydrogen-bonding events that occur when a protein morphs from an unfolded to a folded state.
“A protein must fold properly to become an enzyme or signaling molecule or whatever its function may be — all the many things that proteins do in our bodies,” said University of Illinois Urbana-Champaign chemistry professor Martin Gruebele (MMG), who led the new research with composer and software developer Carla Scaletti.
Misfolded proteins contribute to Alzheimer’s disease, Parkinson’s disease, cystic fibrosis and other disorders. To better understand how this process goes awry, scientists must first determine how a string of amino acids shape-shifts into its final form in the watery environment of the cell. The actual transformations occur very fast, “somewhere between 70 nanoseconds and two microseconds,” Gruebele said.
Hydrogen bonds are relatively weak attractions that align atoms located on different amino acids in the protein. A folding protein will form a series of hydrogen bonds internally and with the water molecules that surround it. In the process, the protein wiggles into countless potential intermediate conformations, sometimes hitting a dead-end and backtracking until it stumbles onto a different path.
The researchers wanted to map the time sequence of hydrogen bonds that occur as the protein folds. But their visualizations could not capture these complex events.
“There are literally tens of thousands of these interactions with water molecules during the short passage between the unfolded and folded state,” Gruebele said.
So the researchers turned to data sonification, a method for converting their molecular data into sounds so that they could “hear” the hydrogen bonds forming. To accomplish this, Scaletti wrote a software program that assigned each hydrogen bond a unique pitch. Molecular simulations generated the essential data, showing where and when two atoms were in the right position in space — and close enough to one another — to hydrogen bond. If the correct conditions for bonding occurred, the software program played a pitch corresponding to that bond. Altogether, the program tracked hundreds of thousands of individual hydrogen-bonding events in sequence.
Numerous studies suggest that audio is processed roughly twice as fast as visual data in the human brain, and humans are better able to detect and remember subtle differences in a sequence of sounds than if the same sequence is represented visually, Scaletti said.
“In our auditory system, we’re really very attuned to small differences in frequency,” she said. “We use frequencies and combinations of frequencies to understand speech, for example.”
A protein spends most of its time in the folded state, so the researchers also came up with a “rarity” function to identify when the rare, fleeting moments of folding or unfolding took place.
The resulting sounds gave them insight into the process, revealing how some hydrogen bonds seem to speed up folding while others appear to slow it. They characterized these transitions, calling the fastest “highway,” the slowest “meander,” and the intermediate ones “ambiguous.”
Including the water molecules in the simulations and hydrogen-bonding analysis was essential to understanding the process, Gruebele said.
“Half of the energy from a protein-folding reaction comes from the water and not from the protein,” he said. “We really learned by doing sonification how water molecules settle into the right place on the protein and how they help the protein conformation change so that it finally becomes folded.”
While hydrogen bonds are not the only factor contributing to protein folding, these bonds often stabilize a transition from one folded state to another, Gruebele said. Other hydrogen bonds may temporarily impede proper folding. For example, a protein may get hung up in a repeating loop that involves one or more hydrogen bonds forming, breaking and forming again — until the protein eventually escapes from this cul de sac to continue its journey to its most stable folded state.
“Unlike the visualization, which looks like a total random mess, you actually hear patterns when you listen to this,” Gruebele said. “This is the stuff that was impossible to visualize but it’s easy to hear.”
The National Science Foundation, National Institutes of Health and Symbolic Sound Corporation supported this research.
Gruebele also is a professor in the Beckman Institute for Advanced Science and Technology.